Development and validation of a predictive scoring system for post-transarterial chemoembolization pain management in liver cancer patients
Highlight box
Key findings
• Identification of pain-related factors: the study identified factors associated with postoperative pain after transarterial chemoembolization (TACE) in liver cancer patients. Factors like tumor quantity, tumor size, microsphere volume, and operation time were found to be significant.
• Development of a predictive scoring system: a novel scoring system was developed to predict the likelihood of moderate to severe postoperative pain following TACE. This system was based on multivariate analysis of the identified factors.
What is known and what is new?
• Postoperative pain in liver cancer treatment: in the context of liver cancer treatment, particularly TACE, postoperative pain is a common and significant issue. Managing this pain effectively is crucial for patient comfort, treatment adherence, and overall outcomes.
• Development of a specific predictive model: this study contributes significantly by developing a specific predictive scoring system for postoperative pain following TACE in liver cancer patients. It goes beyond general pain management principles and offers a tailored approach.
What is the implication, and what should change now?
• Enhanced patient care: by predicting the likelihood of moderate to severe pain, healthcare providers can pre-emptively tailor pain management strategies for each patient. This personalized approach can improve patient comfort, recovery, and overall satisfaction with their care.
• Adoption of the scoring system: clinics and hospitals specializing in liver cancer treatment, particularly those using TACE, should consider integrating this scoring system into their preoperative assessment protocols.
Introduction
Hepatocellular carcinoma (HCC) is currently considered the seventh most common cancer and the second leading cause of cancer mortality (1,2). Transarterial chemoembolization (TACE) has become the standard treatment for patients clinically diagnosed with intermediate and advanced liver cancer who are unable to receive curative intent treatment (3).
Effective management of postoperative pain following TACE is crucial for liver cancer patients (4), as it significantly influences their recovery, treatment adherence, and overall quality of life. Despite advances in pain assessment and management, there remains a notable gap in predictive tools that can accurately forecast pain levels post-TACE, thereby enabling preemptive and more tailored pain management strategies (4-6).
The conventional approach to pain evaluation, predominantly relying on subjective scales such as the 11-point numerical rating scale (NRS-11) (7), often fails to capture the complexity and variability of pain experienced by liver cancer patients undergoing TACE. This inadequacy underscores the urgent need for a more sophisticated and objective predictive model that incorporates specific clinical parameters influencing pain outcomes post-TACE (8,9).
The current study addresses this unmet need by proposing the development and validation of a novel scoring system aimed at predicting moderate to severe post-TACE pain. This initiative is not merely an academic exercise but a clinically relevant endeavor that seeks to bridge the gap between generic pain assessment tools and the need for a more nuanced understanding of pain dynamics in the context of liver cancer treatment.
Moreover, the pursuit of such a predictive model is justified by the potential to enhance patient care through proactive pain management. By accurately identifying patients at higher risk of experiencing significant postoperative pain, healthcare providers can customize pain management plans, thereby improving patient comfort, reducing the reliance on reactive pain interventions, and potentially shortening hospital stays.
In summary, our research is grounded in the clinical imperative to improve pain management in liver cancer patients post-TACE. By addressing the limitations of current pain assessment methods and introducing a tailored predictive tool, this study aims to make a meaningful contribution to the field of pain management and liver cancer care, ultimately enhancing patient outcomes and satisfaction. We present this article in accordance with the TRIPOD reporting checklist (available at https://jgo.amegroups.com/article/view/10.21037/jgo-24-2/rc).
Methods
Study design
From January 2019 to December 2020, patients diagnosed with HCC who received their first TACE treatment session at our institution (Department of Hepatobiliary Surgery, the Second Affiliated Hospital of Chongqing Medical University, Chongqing, China) were included in this retrospective study. The total cohort was randomly divided into training cohort and verification cohort in a 2:1 ratio. The study was reviewed and approved by the Ethics Committee of the Second Affiliated Hospital of Chongqing Medical University (No. protocol code 2022-38 and date of approval 2022-05-08). The data are collected retrospectively and anonymously, so the requirement for informed consent was waived by the ethics committee. The study was conducted in accordance with the Declaration of Helsinki (as revised in 2013).
The inclusion criterion was patients who underwent their first TACE session for the treatment of liver cancer. The diagnosis of liver cancer was primarily based on laboratory examinations and imaging data. Patients who had previously undergone TACE, or those with decompensated cirrhosis, serious cardiovascular and cerebrovascular diseases, renal insufficiency or dysfunction, preoperative infection (such as biliary tract infection, lung infection, urinary system infection, etc.), and a completely occluded portal vein with no collateral blood supply, were excluded from this study.
Pain level scoring
Postoperative pain levels were obtained using the validated, verbally administered, NRS-11 (0 to 10 points) (9,10). At 24 hours before surgery, the nurse educated the patient on pain levels and educated the patient on how to use the NRS-11. Pain scores, based on the patient’s self-assessment, were incorporated into the nursing routine, monitored, and recorded along with vital signs. After surgery, the patient’s pain level was assessed within 24 hours. The score was obtained before any treatments were administered. Using the extracted pain scores of each patient, the participants were divided into two groups: the low score group (≤5, no pain or slight pain) and the high score group (>5, moderate to severe pain).
For the scores between 1 and 3, the patient was taught to relieve pain through distraction. For the scores between 4 and 6, the patient was prescribed a COX-2 inhibitor. If the pain score was ≥7, the doctor would modify the analgesic plan, often to include tramadol hydrochloride, dexocine, or pethidine hydrochloride.
TACE procedures
For femoral artery approach, the right femoral artery was punctured using the Seldinger puncture procedure through a 5-F puncture sheath (Cook Medical, Bloomington, IN, USA). With the use of a contrast guide wire (150 cm; Cook Medical), a contrast catheter (110 cm; Cook Medical) was inserted to the abdominal trunk, common hepatic artery, or superior mesenteric artery, depending on tumor location. Then, a 2.4-F micro catheter (28mc24130sn; Merit Medical, South Jordan, UT, USA) with the help of a micro guide wire (180 cm, Merit Medical) was introduced into the tumor target vessel for chemoembolization. Following the procedure, the puncture sheath was removed and a right femoral artery electronic compression hemostat (01-GF-A; Rising Medical, Chicago, IL, USA) was utilized to compress and halt bleeding.
For radial artery approach, the puncture site was selected to be approximately 1 cm from the patient’s left hand to the proximal end of the radial process (the radial arterial pulse point). After local anesthesia, the site was punctured using a specialized single-arm needle (PSI-4F-11-018; Merit Medical). The guide wire (LWSTDA35180; Merit Medical) and 4-F vascular sheath (PSI-4F-11-018; Merit Medical) were then inserted and anticoagulant and antispasmodic (heparin 2,000 U + nitroglycerin 200 µg + normal saline 20 mL) medicines were administered. Under fluoroscopy, a specialized 4-F radial artery catheter (PV412538U1; Merit Medical) with the help of a guide wire was inserted into the phrenic artery, celiac trunk artery, common hepatic artery, superior mesenteric artery, and renal artery, depending on tumor location. The micro catheter (2.7f130cm; Merit Medical) was then guided to the target vessel for chemoembolization with the help of a micro guide wire (28mc24150sn; Merit Medical). After the procedure was finished, an arterial electronic compression hemostat (AP-S-180; Rising Medical) was utilized to control the bleeding.
Statistical analysis
The software SPSS 22.0 (IBM Corp., Armonk, NY, USA) and R with “rms” and “nomogramEx” packages were used for statistical analysis. Continuous variables were expressed as mean ± standard deviation (SD), and independent sample t-test was used for comparison between groups. Classification variables were expressed in terms of the number of cases and the corresponding proportion, and Fisher’s test was used for comparison between groups. The sample size was estimated with the method of events per variable (EPV) (10) and the sample size was enough in this study. Logistic regression analysis was used to determine the risk factors for pain level after TACE treatment, and variables with P<0.1 obtained from univariate analysis were included in multivariate analysis to build a predictive model. A nomogram was drawn based on the prediction model. The area under the receiver operating characteristic (ROC) curve (AUC) was used to assess the predictive performance of various indicators for postoperative pain. AUC values vary between 0.5 and 1, where 0.5 represents a bad diagnostic test and 1 represents an excellent diagnostic test (11). The Youden index was used to judge the best nomogram score. The Hosmer-Lemeshow (HL) test and calibration plot were used to evaluate the consistency between the predicted and actual probability. To illustrate the clinical relevance of the nomogram, decision curves were drawn to quantify the net benefit for patients at different threshold probabilities. All tests were two-tailed and a P value <0.05 was considered statistically significant.
Results
Demographic and clinical data
A total of 255 patients who underwent initial TACE at our institution were screened for this study. For our study, the random number seed 20210101 was set, and the total cohort was randomly divided into training cohort (n=170) and verification cohort (n=85) in a 2:1 ratio. The general characteristics are shown in Table 1.
Table 1
Variables | Total (n=255) | Training cohort (n=170) | Validation cohort (n=85) | P value |
---|---|---|---|---|
Sex | 0.094 | |||
Male | 205 (80.39) | 142 (83.53) | 63 (74.12) | |
Female | 50 (19.61) | 28 (16.47) | 22 (25.88) | |
Age (years) | 56±13 | 56±12 | 55±13 | 0.768 |
Child-Pugh grade | 0.065 | |||
A | 218 (85.49) | 151 (88.82) | 67 (78.82) | |
B | 35 (13.73) | 18 (10.59) | 17 (20.00) | |
C | 2 (0.78) | 1 (0.59) | 1 (1.18) | |
Tumor number | 0.888 | |||
≤3 | 124 (48.63) | 83 (48.82) | 41 (48.24) | |
>3 | 104 (40.78) | 68 (40.00) | 36 (42.35) | |
Tumor size of the largest tumor (mm) | 68.04±46.38 | 64.77±41.96 | 74.41±53.69 | 0.138 |
BCLC stage | 0.076 | |||
A | 52 (20.39) | 37 (21.76) | 15 (17.65) | |
B | 32 (12.55) | 26 (15.29) | 6 (7.06) | |
C | 141 (55.29) | 89 (52.35) | 52 (61.18) | |
D | 1 (0.39) | 0 | 1 (1.18) | |
AFP level (ng/mL) | 0.482 | |||
≤400 | 163 (63.92) | 106 (62.35) | 57 (67.06) | |
>400 | 86 (33.73) | 60 (35.29) | 26 (30.59) | |
Preoperative ALT (U/L) | 49.34±45.48 | 46.85±49.41 | 54.36±35.98 | 0.216 |
Preoperative AST(U/L) | 69.87±125.31 | 61.67±85.17 | 86.27±180.22 | 0.235 |
Preoperative TBIL (μmol/L) | 25.21±108.10 | 18.30±27.59 | 39.02±183.08 | 0.302 |
TACE routes | 0.894 | |||
Radial artery | 146 (57.25) | 98 (57.65) | 48 (56.47) | |
Femoral artery | 109 (42.74) | 72 (42.35) | 37 (43.53) | |
Superselective TACE | 0.447 | |||
Yes | 247 (96.86) | 166 (97.65) | 81 (95.29) | |
No | 8 (3.14) | 4 (2.35) | 4 (4.71) | |
Lipiodol (mL) | 10.07±4.13 | 10.13±4.14 | 9.95±4.12 | 0.748 |
Microspheres volume (mL) | 3.6±5.6 | 3.9±6.0 | 3.0±4.6 | 0.216 |
Pirarubicin (mL) | 37.92±19.70 | 37.59±19.51 | 38.59±20.19 | 0.703 |
Lobaplatin (mL) | 17.54±19.96 | 16.88±19.80 | 18.85±20.35 | 0.460 |
NRS-11 score | 0.507 | |||
≤5 | 203 (79.61) | 138 (81.18) | 65 (76.47) | |
>5 | 51 (20.00) | 32 (18.82) | 19 (22.35) | |
Operation time (min) | 86.87±38.03 | 86.87±38.03 | 83.15±32.79 | 0.443 |
Data are presented as n (%) or mean ± SD. BCLC, Barcelona Clinic Liver Cancer; AFP, alpha-fetoprotein; ALT, alanine aminotransferase; AST, aspartate aminotransferase; TBIL, total bilirubin; TACE, transarterial chemoembolization; NRS-11, 11-point numerical rating scale (0 to 10 points); SD, standard deviation.
The patients in this study were 80.39% male, with an average age of 56 years. The liver function of most patients was classified as Child-Pugh A (85.49%); 48.63% had three or fewer tumors, whereas 40.78% had more than three tumors. The average diameter of the largest tumor was 68.04 mm, and 62.8% of patients had tumors diagnosed either as Barcelona Clinic Liver Cancer (BCLC) stage C or D. The preoperative levels of alpha-fetoprotein (AFP), aspartate aminotransferase (AST), alanine aminotransferase (ALT), and total bilirubin (TBIL) of the patients are described in Table 1. In this study, 146 patients (57.25%) were treated with TACE through radial artery approach to alleviate postoperative discomfort induced by limb immobilization. Furthermore, most participants received superselective embolization, with only eight patients having no obvious tumor arteries therefore undergoing non-superselective embolization.
Before TACE, all cases had no pain reported. After TACE, we found that 20.1% of cases experienced moderate to severe pain. Personalized analgesic therapy based on the recommendations of members of a specialized pain team was implemented, and after appropriate pain management was attained using oral analgesics alone, the patients’ pain was relieved.
Univariate analysis and multivariate analysis in training cohort
In the training cohort, the univariate analysis found a potential correlation between tumor number [odds ratio (OR), 2.13; 95% confidence interval (CI): 0.94–4.81], tumor size of the largest tumor (OR, 1.01; 95% CI: 1.00–1.02), BCLC stage (OR, 3.72; 95% CI: 1.04–13.32), microspheres volume (OR, 1.05; 95% CI: 0.99–1.12), operation time (OR, 1.01; 95% CI: 1.00–1.02), and postoperative pain levels (all P<0.1). Since the BCLC stage is associated with tumor number and tumor size of the largest tumor, the multivariate analysis only included tumor number, tumor size of the largest tumor, microspheres volume, and operation time. It was found that tumor size of the largest tumor (OR, 1.01; 95% CI: 1.00–1.02) was an independent risk factor for moderate to severe pain levels after TACE. The results of the univariate and multivariate analysis are shown in Table 2.
Table 2
Variables | Univariable analysis | Multivariable analysis | |||
---|---|---|---|---|---|
OR (95% CI) | P value | OR (95% CI) | P value | ||
Sex | |||||
Male/female | 0.82 (0.30–2.23) | 0.700 | |||
Age (years) | 1.01 (0.98–1.04) | 0.484 | |||
Child-Pugh grade | |||||
A | 1 (reference) | ||||
B | 1.26 (0.38–4.10) | 0.707 | |||
C | 0.00 (0.00–NA) | >0.99 | |||
Tumor number | |||||
≤3 | 1 (reference) | 1 (reference) | |||
>3 | 2.13 (0.94–4.81) | 0.069 | 2.02 (0.84–4.84) | 0.115 | |
Tumor size of the largest tumor (mm) | 1.01 (1.00–1.02) | 0.007 | 1.01 (1.00–1.02) | 0.032 | |
BCLC stage | |||||
A | 1 (reference) | ||||
B | 2.70 (0.58–12.48) | 0.204 | |||
C | 3.72 (1.04–13.32) | 0.043 | |||
AFP level (ng/mL) | |||||
>400/≤400 | 1.71 (0.77–3.81) | 0.188 | |||
Preoperative ALT (U/L) | 1.00 (0.99–1.01) | 0.530 | |||
Preoperative AST (U/L) | 1.00 (1.00–1.01) | 0.568 | |||
Preoperative TBIL (μmol/L) | 1.00 (0.99–1.02) | 0.804 | |||
TACE procedures | |||||
TRA/TFA | 1.80 (0.79–4.07) | 0.162 | |||
Superselective TACE | |||||
Yes/no | 3.86E+8 (0–NA) | >0.99 | |||
Lipiodol (mL) | 1.06 (0.97–1.15) | 0.208 | |||
Microspheres volume (mL) | 1.05 (0.99–1.12) | 0.090 | 1.03 (0.97–1.10) | 0.372 | |
Pirarubicin (mL) | 1.01 (0.99–1.03) | 0.329 | |||
Lobaplatin (mL) | 1.00 (0.98–1.02) | 0.844 | |||
Operation time (min) | 1.01 (1.00–1.02) | 0.044 | 1.01 (1.00–1.02) | 0.117 |
OR, odds ratio; CI, confidence interval; NA, not available; BCLC, Barcelona Clinic Liver Cancer; AFP, alpha-fetoprotein; ALT, alanine aminotransferase; AST, aspartate aminotransferase; TBIL, total bilirubin; TACE, transarterial chemoembolization; TRA, transradial access; TFA, transfemoral access.
Construction of prediction model and prediction efficiency analysis
The prediction model of postoperative pain levels after TACE consists of tumor number, tumor size of the largest tumor, microsphere volume, and operation time and can be expressed using the following formula: logit(P) = 0.702 × tumor number + 0.011 × tumor size of the largest tumor + 0.028 × microsphere volume + 0.008 × operation time-3.447. When these variables were applied, the AUC for postoperative moderate to severe pain was 0.71 (95% CI: 0.62–0.80), suggesting that the model has a high degree of differentiation (Figure 1A). The HL test was performed to analyze the calibration of the prediction model, and it showed no statistically significant difference between the predicted and actual values, indicating a high calibration ability of the prediction model (HL test χ2=10.963, P=0.204; Figure 1B).
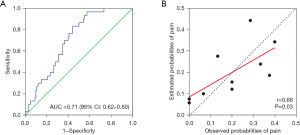
Validation of the prediction model
The validation cohort was used for verification. When utilizing the previously identified four indicators, the AUC for moderate to severe postoperative pain was 0.74 (95% CI: 0.62–0.85), indicating that this model has a high degree of differentiation (Figure 2A). The HL test was again used to evaluate the calibration of this prediction model, and showed no statistically significant difference between the predicted and actual values of the model, suggesting that the novel prediction model had high calibration ability (HL test χ2=8.516, P=0.385; Figure 2B).
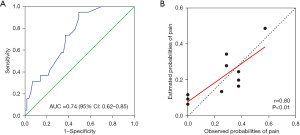
Predictive effect of nomogram on the risk of postoperative pain after TACE
We developed a nomogram of the novel prediction model using the training cohort for better clinical application (Figure 3). The scores for each patient were calculated based on the tumor number, tumor size of the largest tumor, microsphere volume, and operation time, with a cut-off value of 72.90 set as a threshold. Patients in the training and validation cohorts were divided into two groups: high-risk and low-risk, according to this threshold. In the training cohort, the incidence rate of post-TACE pain was higher in the high-risk group (29/98, 29.59%) than in the low-risk group (1/52, 1.92%) (P=0.001). Similarly, in the validation cohort, the incidence rate was higher in the high-risk group (19/57, 33.33%) than in the low-risk group (0/19, 0%), with a statistically significant difference (P=0.002). Furthermore, the decision curve analysis indicated that the model is capable of accurately predicting postoperative pain (Figure 4).
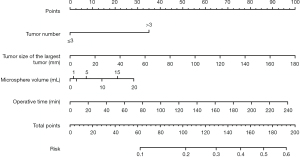
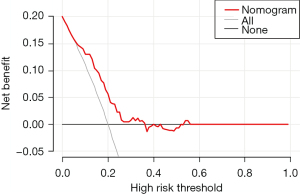
Discussion
TACE serves as a pivotal treatment for intermediate and advanced liver cancer, significantly improving survival rates and potentially enabling some patients for curative interventions (12,13). Our study builds on this foundation by addressing the management of post-embolization syndrome (PES), a notable side effect of TACE, through a novel predictive scoring system for post-TACE pain in liver cancer patients (14-16).
We identified that factors such as tumor number, size, microsphere volume, and operative time are closely associated with the occurrence of post-TACE pain (17-19). These findings are in line with previous studies, underscoring the relationship between tumor characteristics and post-treatment outcomes. Notably, our work advances the understanding of PES by distinguishing it clearly as a treatment side effect, rather than a complication, and by providing a quantifiable means to predict its severity (20,21).
Our predictive model, substantiated by a robust multivariate analysis, offers a practical tool for clinicians to assess the risk of significant pain post-TACE, thereby facilitating tailored perioperative pain management strategies. This approach not only enhances patient comfort but also integrates seamlessly with the principles of enhanced recovery after surgery (ERAS) (22-24), contributing to improved postoperative care and potentially reducing healthcare costs.
Despite the promising implications of our study, it is important to acknowledge its limitations, including the sample size and the retrospective design, which may affect the generalizability of our findings. Future research should aim for external validation of our predictive model and explore the prospective application in diverse clinical settings.
Conclusions
Our study presents a significant stride towards optimizing post-TACE patient care by enabling the preemptive identification of individuals at higher risk for severe pain, thereby refining pain management protocols and supporting the broader adoption of ERAS principles in liver cancer treatment.
Acknowledgments
Funding: The study was funded by
Footnote
Reporting Checklist: The authors have completed the TRIPOD reporting checklist. Available at https://jgo.amegroups.com/article/view/10.21037/jgo-24-2/rc
Data Sharing Statement: Available at https://jgo.amegroups.com/article/view/10.21037/jgo-24-2/dss
Peer Review File: Available at https://jgo.amegroups.com/article/view/10.21037/jgo-24-2/prf
Conflicts of Interest: All authors have completed the ICMJE uniform disclosure form (available at https://jgo.amegroups.com/article/view/10.21037/jgo-24-2/coif). The authors have no conflicts of interest to declare.
Ethical Statement: The authors are accountable for all aspects of the work in ensuring that questions related to the accuracy or integrity of any part of the work are appropriately investigated and resolved. The study was reviewed and approved by the Institutional Review Board and the Ethics Committee of the Second Affiliated Hospital of Chongqing Medical University (No. protocol code 2022-38 and date of approval 2022-05-08). The data are collected retrospectively and anonymously, so the requirement for informed consent was waived by the ethics committee. The study was conducted in accordance with the Declaration of Helsinki (as revised in 2013).
Open Access Statement: This is an Open Access article distributed in accordance with the Creative Commons Attribution-NonCommercial-NoDerivs 4.0 International License (CC BY-NC-ND 4.0), which permits the non-commercial replication and distribution of the article with the strict proviso that no changes or edits are made and the original work is properly cited (including links to both the formal publication through the relevant DOI and the license). See: https://creativecommons.org/licenses/by-nc-nd/4.0/.
References
- Sung H, Ferlay J, Siegel RL, et al. Global Cancer Statistics 2020: GLOBOCAN Estimates of Incidence and Mortality Worldwide for 36 Cancers in 185 Countries. CA Cancer J Clin 2021;71:209-49. [Crossref] [PubMed]
- McGlynn KA, Petrick JL, El-Serag HB. Epidemiology of Hepatocellular Carcinoma. Hepatology 2021;73:4-13. [Crossref] [PubMed]
- Reig M, Forner A, Rimola J, et al. BCLC strategy for prognosis prediction and treatment recommendation: The 2022 update. J Hepatol 2022;76:681-93. [Crossref] [PubMed]
- Lyu N, Kong Y, Li X, et al. Effect and Safety of Prophylactic Parecoxib for Pain Control of Transarterial Chemoembolization in Liver Cancer: A Single-Center, Parallel-Group, Randomized Trial. J Am Coll Radiol 2022;19:61-70. [Crossref] [PubMed]
- Mason MC, Massarweh NN, Salami A, et al. Post-embolization syndrome as an early predictor of overall survival after transarterial chemoembolization for hepatocellular carcinoma. HPB (Oxford) 2015;17:1137-44. [Crossref] [PubMed]
- Lu H, Zheng C, Liang B, et al. Mechanism and risk factors of nausea and vomiting after TACE: a retrospective analysis. BMC Cancer 2021;21:513. [Crossref] [PubMed]
- Lee HJ, Cho Y, Joo H, et al. Comparative study of verbal rating scale and numerical rating scale to assess postoperative pain intensity in the post anesthesia care unit: A prospective observational cohort study. Medicine (Baltimore) 2021;100:e24314. [Crossref] [PubMed]
- Hartrumpf KJ, Marquardt S, Werncke T, et al. Quality of life in patients undergoing repetitive TACE for the treatment of intermediate stage HCC. J Cancer Res Clin Oncol 2018;144:1991-9. [Crossref] [PubMed]
- Guo JG, Zhao LP, Rao YF, et al. Novel multimodal analgesia regimen improves post-TACE pain in patients with hepatocellular carcinoma. Hepatobiliary Pancreat Dis Int 2018;17:510-6. [Crossref] [PubMed]
- Peduzzi P, Concato J, Kemper E, et al. A simulation study of the number of events per variable in logistic regression analysis. J Clin Epidemiol 1996;49:1373-9. [Crossref] [PubMed]
- Cook NR. Use and misuse of the receiver operating characteristic curve in risk prediction. Circulation 2007;115:928-35. [Crossref] [PubMed]
- Zhong BY, Jin ZC, Chen JJ, et al. Role of Transarterial Chemoembolization in the Treatment of Hepatocellular Carcinoma. J Clin Transl Hepatol 2023;11:480-9. [Crossref] [PubMed]
- Hu J, Mao H, He Y. Systematic review and meta-analysis of the efficacy and safety of high-intensity focused ultrasound combined with transarterial chemoembolization and transarterial chemoembolization alone in the treatment of liver cancer. Transl Cancer Res 2022;11:1678-88. [Crossref] [PubMed]
- Roehlen N, Stoehr F, Müller L, et al. Prediction of postembolization syndrome after transarterial chemoembolization of hepatocellular carcinoma and its impact on prognosis. Hepatol Commun 2023;7:e0252. [Crossref] [PubMed]
- Blackburn H, West S. Management of Postembolization Syndrome Following Hepatic Transarterial Chemoembolization for Primary or Metastatic Liver Cancer. Cancer Nurs 2016;39:E1-E18. [Crossref] [PubMed]
- Wang TC, Zhang ZS, Xiao YD. Determination of Risk Factors for Pain After Transarterial Chemoembolization with Drug-Eluting Beads for Hepatocellular Carcinoma. J Pain Res 2020;13:649-56. [Crossref] [PubMed]
- Du QQ, Liang M, Jiang B, et al. Incidence and predictors of abdominal pain after transarterial chemoembolization of hepatocellular carcinoma: a single-center retrospective study. Eur J Oncol Nurs 2023;66:102355. [Crossref] [PubMed]
- Benzakoun J, Ronot M, Lagadec M, et al. Risks factors for severe pain after selective liver transarterial chemoembolization. Liver Int 2017;37:583-91. [Crossref] [PubMed]
- Cohen MJ, Bloom AI, Barak O, et al. Trans-arterial chemo-embolization is safe and effective for very elderly patients with hepatocellular carcinoma. World J Gastroenterol 2013;19:2521-8. [Crossref] [PubMed]
- Wu Y, Yang G, Li Z, et al. Conservative treatment of hepatic portal vein gas after transarterial chemoembolization treatment for liver metastasis of postoperative esophageal cancer: a case report. J Gastrointest Oncol 2023;14:1166-74. [Crossref] [PubMed]
- Dhand S, Gupta R. Hepatic transcatheter arterial chemoembolization complicated by postembolization syndrome. Semin Intervent Radiol 2011;28:207-11. [Crossref] [PubMed]
- Mumtaz K, Patel N, Modi RM, et al. Trends and outcomes of transarterial chemoembolization in hepatocellular carcinoma: a national survey. Hepatobiliary Pancreat Dis Int 2017;16:624-30. [Crossref] [PubMed]
- Simpson JC, Bao X, Agarwala A. Pain Management in Enhanced Recovery after Surgery (ERAS) Protocols. Clin Colon Rectal Surg 2019;32:121-8. [Crossref] [PubMed]
- Beverly A, Kaye AD, Ljungqvist O, et al. Essential Elements of Multimodal Analgesia in Enhanced Recovery After Surgery (ERAS) Guidelines. Anesthesiol Clin 2017;35:e115-43. [Crossref] [PubMed]